당신은 주제를 찾고 있습니까 “which class of biological polymer has the greatest functional variety – 003-Biological Polymers“? 다음 카테고리의 웹사이트 https://chewathai27.com/you 에서 귀하의 모든 질문에 답변해 드립니다: https://chewathai27.com/you/blog. 바로 아래에서 답을 찾을 수 있습니다. 작성자 Fundamentals of Biochemistry 이(가) 작성한 기사에는 조회수 19,292회 및 좋아요 106개 개의 좋아요가 있습니다.
A question is with class of biological polymer polymer has the greatest variety functional, greatest functional variety. So your first option is policy, great policy rates. And next option is protein proteins and corruption is DNA the oxy ribonucleic acid. And last option is RNA ribonucleic acid.Proteins. Proteins are one of the most abundant organic molecules in living systems and have the most diverse range of functions of all macromolecules.There are four basic kinds of biological macromolecules: carbohydrates, lipids, proteins, and nucleic acids. These polymers are composed of different monomers and serve different functions.
- DNA. DNA (deoxyribonucleic acid) is a polymer which is essential for life. …
- Proteins. Proteins are biological polymers made inside cells. …
- Starch and cellulose. Starch and cellulose are biological polymers which are made by plants.
which class of biological polymer has the greatest functional variety 주제에 대한 동영상 보기
여기에서 이 주제에 대한 비디오를 시청하십시오. 주의 깊게 살펴보고 읽고 있는 내용에 대한 피드백을 제공하세요!
d여기에서 003-Biological Polymers – which class of biological polymer has the greatest functional variety 주제에 대한 세부정보를 참조하세요
Brief review of types of bio polymers
which class of biological polymer has the greatest functional variety 주제에 대한 자세한 내용은 여기를 참조하세요.
Which class of biological polymer has the greatest … – Study.com
Answer to: Which of biological polymer has the greatest functional variety? 1) DNA 2) polysacchares 3) proteins 4) RNA.
Source: study.com
Date Published: 10/27/2022
View: 2723
Which class of biological polymer has the … – AnswerPrime.com
Which of biological polymer has the greatest functional variety? … Approximately 32 different monomeric carbohydrate subunits are found in …
Source: answerprime.com
Date Published: 4/12/2022
View: 9876
Which class of biological polymer has the greatest functional …
Which of biological polymer has the greatest functional variety General. 760 students attemted this question.
Source: mcqpoint.com
Date Published: 9/7/2022
View: 8733
Chapter 5 Flashcards – Easy Notecards
Which of biological polymer has the greatest functional variety? A) polysacchares. B) proteins. C) DNA D) RNA. Answer: B.
Source: www.easynotecards.com
Date Published: 12/12/2022
View: 1227
What are the 4 biological polymers? – Book Vea
… organic molecules in living systems and have the most diverse …
Source: bookvea.com
Date Published: 3/23/2022
View: 9005
주제와 관련된 이미지 which class of biological polymer has the greatest functional variety
주제와 관련된 더 많은 사진을 참조하십시오 003-Biological Polymers. 댓글에서 더 많은 관련 이미지를 보거나 필요한 경우 더 많은 관련 기사를 볼 수 있습니다.
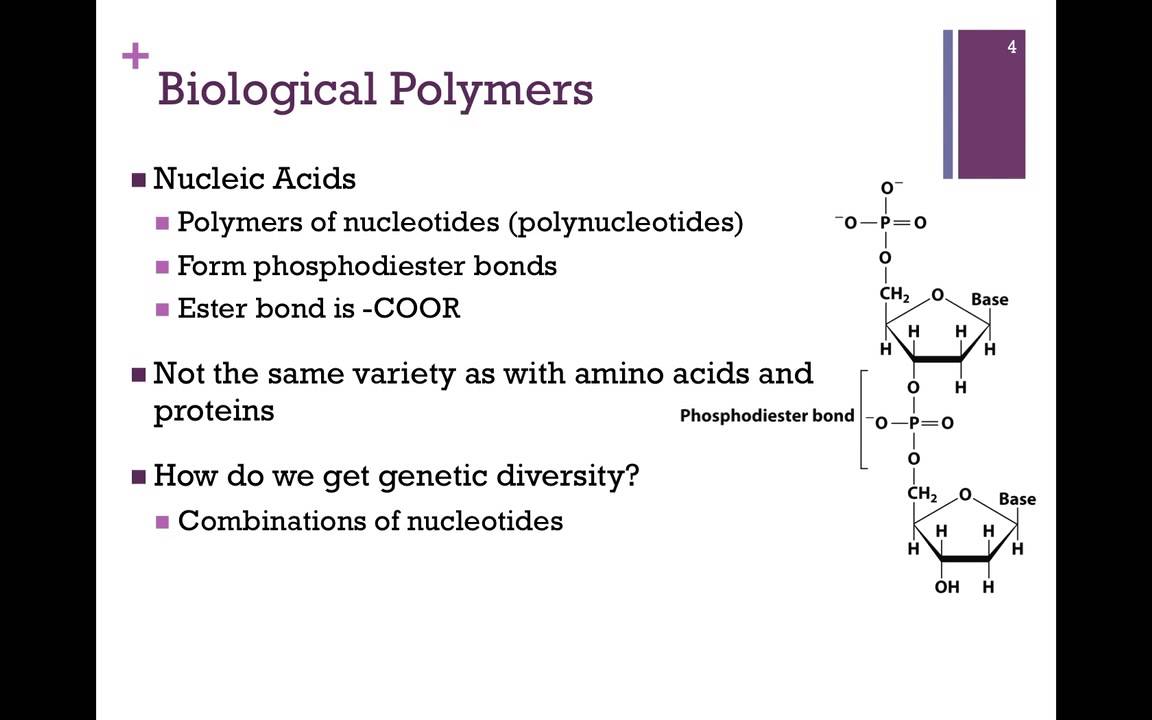
주제에 대한 기사 평가 which class of biological polymer has the greatest functional variety
- Author: Fundamentals of Biochemistry
- Views: 조회수 19,292회
- Likes: 좋아요 106개
- Date Published: 2014. 6. 10.
- Video Url link: https://www.youtube.com/watch?v=ToJ2zlRnJto
Which of the biological polymers has the most diverse functions?
Proteins. Proteins are one of the most abundant organic molecules in living systems and have the most diverse range of functions of all macromolecules.
What are the 4 types of biological polymers?
There are four basic kinds of biological macromolecules: carbohydrates, lipids, proteins, and nucleic acids. These polymers are composed of different monomers and serve different functions.
What are the three major biological polymers?
There are three main classes of biopolymers, classified according to the monomers used and the structure of the biopolymer formed: polynucleotides, polypeptides, and polysaccharides. Polynucleotides, such as RNA and DNA, are long polymers composed of 13 or more nucleotide monomers.
What are the biologically important polymers?
- DNA. DNA (deoxyribonucleic acid) is a polymer which is essential for life. …
- Proteins. Proteins are biological polymers made inside cells. …
- Starch and cellulose. Starch and cellulose are biological polymers which are made by plants.
Which biomolecule is most important?
Proteins. Proteins are the primary building materials of the body. Your hair, skin, muscles, and organs are composed mostly of proteins. Proteins are strong yet flexible, and they have a complex 3-D structure.
What biomolecule is the most abundant?
The main component of plant cell walls is cellulose, which is the most abundant biomolecule on earth.
What are the four classes of large biological molecules please briefly explain their cellular function?
biomolecule, also called biological molecule, any of numerous substances that are produced by cells and living organisms. Biomolecules have a wide range of sizes and structures and perform a vast array of functions. The four major types of biomolecules are carbohydrates, lipids, nucleic acids, and proteins.
What are the two categories of polymers?
- thermosetting plastic or thermoset.
- thermoforming plastic or thermoplastic.
Which class of biological molecules does not include polymers?
Just remember that lipids are one of the four main types of large biological molecules, but that they don’t generally form polymers.
What are the four general classes of biological macromolecules?
A large, organic molecule such as carbohydrates, lipids, proteins, and nucleic acids.
What is the most important macromolecule?
Nucleic acids are the most important macromolecules for the continuity of life. They carry the genetic blueprint of a cell and carry instructions for the functioning of the cell.
Which of the following is the major function of carbohydrates?
Alongside fat and protein, carbohydrates are one of the three macronutrients in our diet with their main function being to provide energy to the body.
Which two functional groups do the monomers need to make a polypeptide?
Amino acids are molecules which have at least two functional groups. All amino acids contain the -NH 2 group and also the carboxylic acid group -COOH. Amino acids are polymerised in cells to make polypeptides and proteins .
How many functional groups do monomers in condensation polymerisation have?
A condensation polymer is a polymer formed by condensation reactions. Monomers of condensation polymers must contain two functional groups so that each monomer can link up with two other monomers.
How many functional groups do amino acids have?
Carboxyl and amino groups are always found in amino acids. The amino group consists of an atom of nitrogen bound to hydrogen atoms by single bonds. An organic compound is considered an amine that contains an amino group.
How do organic polymers contribute to biological diversity?
Explain how organic polymers contribute to biological diversity: Polymers make up DNA and proteins, so any variation in polymers will vary DNA and proteins, as well. Distinguish between monosaccharides and disaccharides: Monosaccharides are simple (single) sugars and have molecular formulas that are multiples of CH2O.
What are the major functions of proteins?
- Growth and Maintenance. Share on Pinterest. …
- Causes Biochemical Reactions. …
- Acts as a Messenger. …
- Provides Structure. …
- Maintains Proper pH. …
- Balances Fluids. …
- Bolsters Immune Health. …
- Transports and Stores Nutrients.
What are the distinguishing characteristics of carbohydrates?
INTRODUCTION. Carbohydrates represent a broad group of substances which include the sugars, starches, gums and celluloses. The common attributes of carbohydrates are that they contain only the elements carbon, hydrogen and oxygen, and that their combustion will yield carbon dioxide plus one or more molecules of Water.
What distinguishes an amino acid from a carbohydrate?
Answer and Explanation: The difference between an amino acid and a carbohydrate is that amino acids are the building blocks of proteins, while carbohydrates are sugar and… See full answer below.
SOLVED:Which class of biological polymer has the greatest variety? functional polysaccharides proteins DNA RNA
The Elements of Life
In biology, the elements of life are the essential building blocks that make up living things. They are carbon, hydrogen, nitrogen, oxygen, phosphorus, and sulfur. The first four of these are the most important, as they are used to construct the molecules that are necessary to make up living cells. These elements form the basic building blocks of the major macromolecules of life, including carbohydrates, lipids, nucleic acids and proteins. Carbon is an important element for all living organisms, as it is used to construct the basic building blocks of life, such as carbohydrates, lipids, and nucleic acids. Even the cell membranes are made of proteins. Carbon is also used to construct the energy-rich molecules adenosine triphosphate (ATP) and guanosine triphosphate (GTP). Hydrogen is used to construct the molecules water and organic compounds with carbon. Hydrogen is also used to construct ATP and GTP. Nitrogen is used to construct the basic building blocks of life, such as amino acids, nucleic acids, and proteins. It is also used to construct ATP and GTP. Oxygen is used to construct the basic building blocks of life, such as carbohydrates, lipids, and nucleic acids. It is also used to construct ATP and GTP. Phosphorus is used to construct the basic building blocks of life, such as carbohydrates, lipids, and nucleic acids.
2.3 Biological Molecules – Concepts of Biology – 1st Canadian Edition
2.3 Biological Molecules
Learning Objectives By the end of this section, you will be able to: Describe the ways in which carbon is critical to life
Explain the impact of slight changes in amino acids on organisms
Describe the four major types of biological molecules
Understand the functions of the four major types of molecules
Watch a video about proteins and protein enzymes.
The large molecules necessary for life that are built from smaller organic molecules are called biological macromolecules. There are four major classes of biological macromolecules (carbohydrates, lipids, proteins, and nucleic acids), and each is an important component of the cell and performs a wide array of functions. Combined, these molecules make up the majority of a cell’s mass. Biological macromolecules are organic, meaning that they contain carbon. In addition, they may contain hydrogen, oxygen, nitrogen, phosphorus, sulfur, and additional minor elements.
Carbon It is often said that life is “carbon-based.” This means that carbon atoms, bonded to other carbon atoms or other elements, form the fundamental components of many, if not most, of the molecules found uniquely in living things. Other elements play important roles in biological molecules, but carbon certainly qualifies as the “foundation” element for molecules in living things. It is the bonding properties of carbon atoms that are responsible for its important role.
Carbon Bonding Carbon contains four electrons in its outer shell. Therefore, it can form four covalent bonds with other atoms or molecules. The simplest organic carbon molecule is methane (CH 4 ), in which four hydrogen atoms bind to a carbon atom. However, structures that are more complex are made using carbon. Any of the hydrogen atoms can be replaced with another carbon atom covalently bonded to the first carbon atom. In this way, long and branching chains of carbon compounds can be made (Figure 2.13 a). The carbon atoms may bond with atoms of other elements, such as nitrogen, oxygen, and phosphorus (Figure 2.13 b). The molecules may also form rings, which themselves can link with other rings (Figure 2.13 c). This diversity of molecular forms accounts for the diversity of functions of the biological macromolecules and is based to a large degree on the ability of carbon to form multiple bonds with itself and other atoms.
Carbohydrates Carbohydrates are macromolecules with which most consumers are somewhat familiar. To lose weight, some individuals adhere to “low-carb” diets. Athletes, in contrast, often “carb-load” before important competitions to ensure that they have sufficient energy to compete at a high level. Carbohydrates are, in fact, an essential part of our diet; grains, fruits, and vegetables are all natural sources of carbohydrates. Carbohydrates provide energy to the body, particularly through glucose, a simple sugar. Carbohydrates also have other important functions in humans, animals, and plants. Carbohydrates can be represented by the formula (CH 2 O) n , where n is the number of carbon atoms in the molecule. In other words, the ratio of carbon to hydrogen to oxygen is 1:2:1 in carbohydrate molecules. Carbohydrates are classified into three subtypes: monosaccharides, disaccharides, and polysaccharides. Monosaccharides (mono- = “one”; sacchar- = “sweet”) are simple sugars, the most common of which is glucose. In monosaccharides, the number of carbon atoms usually ranges from three to six. Most monosaccharide names end with the suffix -ose. Depending on the number of carbon atoms in the sugar, they may be known as trioses (three carbon atoms), pentoses (five carbon atoms), and hexoses (six carbon atoms). Monosaccharides may exist as a linear chain or as ring-shaped molecules; in aqueous solutions, they are usually found in the ring form. The chemical formula for glucose is C 6 H 12 O 6 . In most living species, glucose is an important source of energy. During cellular respiration, energy is released from glucose, and that energy is used to help make adenosine triphosphate (ATP). Plants synthesize glucose using carbon dioxide and water by the process of photosynthesis, and the glucose, in turn, is used for the energy requirements of the plant. The excess synthesized glucose is often stored as starch that is broken down by other organisms that feed on plants. Galactose (part of lactose, or milk sugar) and fructose (found in fruit) are other common monosaccharides. Although glucose, galactose, and fructose all have the same chemical formula (C 6 H 12 O 6 ), they differ structurally and chemically (and are known as isomers) because of differing arrangements of atoms in the carbon chain. Disaccharides (di- = “two”) form when two monosaccharides undergo a dehydration reaction (a reaction in which the removal of a water molecule occurs). During this process, the hydroxyl group (–OH) of one monosaccharide combines with a hydrogen atom of another monosaccharide, releasing a molecule of water (H 2 O) and forming a covalent bond between atoms in the two sugar molecules. Common disaccharides include lactose, maltose, and sucrose. Lactose is a disaccharide consisting of the monomers glucose and galactose. It is found naturally in milk. Maltose, or malt sugar, is a disaccharide formed from a dehydration reaction between two glucose molecules. The most common disaccharide is sucrose, or table sugar, which is composed of the monomers glucose and fructose. A long chain of monosaccharides linked by covalent bonds is known as a polysaccharide (poly- = “many”). The chain may be branched or unbranched, and it may contain different types of monosaccharides. Polysaccharides may be very large molecules. Starch, glycogen, cellulose, and chitin are examples of polysaccharides. Starch is the stored form of sugars in plants and is made up of amylose and amylopectin (both polymers of glucose). Plants are able to synthesize glucose, and the excess glucose is stored as starch in different plant parts, including roots and seeds. The starch that is consumed by animals is broken down into smaller molecules, such as glucose. The cells can then absorb the glucose. Glycogen is the storage form of glucose in humans and other vertebrates, and is made up of monomers of glucose. Glycogen is the animal equivalent of starch and is a highly branched molecule usually stored in liver and muscle cells. Whenever glucose levels decrease, glycogen is broken down to release glucose. Cellulose is one of the most abundant natural biopolymers. The cell walls of plants are mostly made of cellulose, which provides structural support to the cell. Wood and paper are mostly cellulosic in nature. Cellulose is made up of glucose monomers that are linked by bonds between particular carbon atoms in the glucose molecule. Every other glucose monomer in cellulose is flipped over and packed tightly as extended long chains. This gives cellulose its rigidity and high tensile strength—which is so important to plant cells. Cellulose passing through our digestive system is called dietary fiber. While the glucose-glucose bonds in cellulose cannot be broken down by human digestive enzymes, herbivores such as cows, buffalos, and horses are able to digest grass that is rich in cellulose and use it as a food source. In these animals, certain species of bacteria reside in the rumen (part of the digestive system of herbivores) and secrete the enzyme cellulase. The appendix also contains bacteria that break down cellulose, giving it an important role in the digestive systems of ruminants. Cellulases can break down cellulose into glucose monomers that can be used as an energy source by the animal. Carbohydrates serve other functions in different animals. Arthropods, such as insects, spiders, and crabs, have an outer skeleton, called the exoskeleton, which protects their internal body parts. This exoskeleton is made of the biological macromolecule chitin, which is a nitrogenous carbohydrate. It is made of repeating units of a modified sugar containing nitrogen. Thus, through differences in molecular structure, carbohydrates are able to serve the very different functions of energy storage (starch and glycogen) and structural support and protection (cellulose and chitin). Registered Dietitian: Obesity is a worldwide health concern, and many diseases, such as diabetes and heart disease, are becoming more prevalent because of obesity. This is one of the reasons why registered dietitians are increasingly sought after for advice. Registered dietitians help plan food and nutrition programs for individuals in various settings. They often work with patients in health-care facilities, designing nutrition plans to prevent and treat diseases. For example, dietitians may teach a patient with diabetes how to manage blood-sugar levels by eating the correct types and amounts of carbohydrates. Dietitians may also work in nursing homes, schools, and private practices. To become a registered dietitian, one needs to earn at least a bachelor’s degree in dietetics, nutrition, food technology, or a related field. In addition, registered dietitians must complete a supervised internship program and pass a national exam. Those who pursue careers in dietetics take courses in nutrition, chemistry, biochemistry, biology, microbiology, and human physiology. Dietitians must become experts in the chemistry and functions of food (proteins, carbohydrates, and fats). Through the Indigenous Lens (Suzanne Wilkerson and Charles Molnar) I work at Camosun College located in beautiful Victoria, British Columbia with campuses on the Traditional Territories of the Lekwungen and W̱SÁNEĆ peoples. The underground storage bulb of the camas flower shown below has been an important food source for many of the Indigenous peoples of Vancouver Island and throughout the western area of North America. Camas bulbs are still eaten as a traditional food source and the preparation of the camas bulbs relates to this text section about carbohydrates. Most often plants create starch as the stored form of carbohydrate. Some plants, like camas create inulin. Inulin is used as dietary fibre however, it is not readily digested by humans. If you were to bite into a raw camas bulb it would taste bitter and has a gummy texture. The method used by Indigenous peoples to make camas both digestible and tasty is to bake the bulbs slowly for a long period in an underground firepit covered with specific leaves and soil. The heat acts like our pancreatic amylase enzyme and breaks down the long chains of inulin into digestible mono and di-saccharides. Properly baked, the camas bulbs taste like a combination of baked pear and cooked fig. It is important to note that while the blue camas is a food source, it should not be confused with the white death camas, which is particularly toxic and deadly. The flowers look different, but the bulbs look very similar.
Lipids Lipids include a diverse group of compounds that are united by a common feature. Lipids are hydrophobic (“water-fearing”), or insoluble in water, because they are nonpolar molecules. This is because they are hydrocarbons that include only nonpolar carbon-carbon or carbon-hydrogen bonds. Lipids perform many different functions in a cell. Cells store energy for long-term use in the form of lipids called fats. Lipids also provide insulation from the environment for plants and animals. For example, they help keep aquatic birds and mammals dry because of their water-repelling nature. Lipids are also the building blocks of many hormones and are an important constituent of the plasma membrane. Lipids include fats, oils, waxes, phospholipids, and steroids. A fat molecule, such as a triglyceride, consists of two main components—glycerol and fatty acids. Glycerol is an organic compound with three carbon atoms, five hydrogen atoms, and three hydroxyl (–OH) groups. Fatty acids have a long chain of hydrocarbons to which an acidic carboxyl group is attached, hence the name “fatty acid.” The number of carbons in the fatty acid may range from 4 to 36; most common are those containing 12–18 carbons. In a fat molecule, a fatty acid is attached to each of the three oxygen atoms in the –OH groups of the glycerol molecule with a covalent bond. During this covalent bond formation, three water molecules are released. The three fatty acids in the fat may be similar or dissimilar. These fats are also called triglycerides because they have three fatty acids. Some fatty acids have common names that specify their origin. For example, palmitic acid, a saturated fatty acid, is derived from the palm tree. Arachidic acid is derived from Arachis hypogaea, the scientific name for peanuts. Fatty acids may be saturated or unsaturated. In a fatty acid chain, if there are only single bonds between neighboring carbons in the hydrocarbon chain, the fatty acid is saturated. Saturated fatty acids are saturated with hydrogen; in other words, the number of hydrogen atoms attached to the carbon skeleton is maximized. When the hydrocarbon chain contains a double bond, the fatty acid is an unsaturated fatty acid. Most unsaturated fats are liquid at room temperature and are called oils. If there is one double bond in the molecule, then it is known as a monounsaturated fat (e.g., olive oil), and if there is more than one double bond, then it is known as a polyunsaturated fat (e.g., canola oil). Saturated fats tend to get packed tightly and are solid at room temperature. Animal fats with stearic acid and palmitic acid contained in meat, and the fat with butyric acid contained in butter, are examples of saturated fats. Mammals store fats in specialized cells called adipocytes, where globules of fat occupy most of the cell. In plants, fat or oil is stored in seeds and is used as a source of energy during embryonic development. Unsaturated fats or oils are usually of plant origin and contain unsaturated fatty acids. The double bond causes a bend or a “kink” that prevents the fatty acids from packing tightly, keeping them liquid at room temperature. Olive oil, corn oil, canola oil, and cod liver oil are examples of unsaturated fats. Unsaturated fats help to improve blood cholesterol levels, whereas saturated fats contribute to plaque formation in the arteries, which increases the risk of a heart attack. In the food industry, oils are artificially hydrogenated to make them semi-solid, leading to less spoilage and increased shelf life. Simply speaking, hydrogen gas is bubbled through oils to solidify them. During this hydrogenation process, double bonds of the cis-conformation in the hydrocarbon chain may be converted to double bonds in the trans-conformation. This forms a trans-fat from a cis-fat. The orientation of the double bonds affects the chemical properties of the fat. Margarine, some types of peanut butter, and shortening are examples of artificially hydrogenated trans-fats. Recent studies have shown that an increase in trans-fats in the human diet may lead to an increase in levels of low-density lipoprotein (LDL), or “bad” cholesterol, which, in turn, may lead to plaque deposition in the arteries, resulting in heart disease. Many fast food restaurants have recently eliminated the use of trans-fats, and U.S. food labels are now required to list their trans-fat content. Essential fatty acids are fatty acids that are required but not synthesized by the human body. Consequently, they must be supplemented through the diet. Omega-3 fatty acids fall into this category and are one of only two known essential fatty acids for humans (the other being omega-6 fatty acids). They are a type of polyunsaturated fat and are called omega-3 fatty acids because the third carbon from the end of the fatty acid participates in a double bond. Salmon, trout, and tuna are good sources of omega-3 fatty acids. Omega-3 fatty acids are important in brain function and normal growth and development. They may also prevent heart disease and reduce the risk of cancer. Like carbohydrates, fats have received a lot of bad publicity. It is true that eating an excess of fried foods and other “fatty” foods leads to weight gain. However, fats do have important functions. Fats serve as long-term energy storage. They also provide insulation for the body. Therefore, “healthy” unsaturated fats in moderate amounts should be consumed on a regular basis. Phospholipids are the major constituent of the plasma membrane. Like fats, they are composed of fatty acid chains attached to a glycerol or similar backbone. Instead of three fatty acids attached, however, there are two fatty acids and the third carbon of the glycerol backbone is bound to a phosphate group. The phosphate group is modified by the addition of an alcohol. A phospholipid has both hydrophobic and hydrophilic regions. The fatty acid chains are hydrophobic and exclude themselves from water, whereas the phosphate is hydrophilic and interacts with water. Cells are surrounded by a membrane, which has a bilayer of phospholipids. The fatty acids of phospholipids face inside, away from water, whereas the phosphate group can face either the outside environment or the inside of the cell, which are both aqueous. Through the Indigenous Lens For the First peoples of the Pacific Northwest the fat rich fish ooligan, with 20% fat by body weight, was a crucial part of the diet of several First Nations. Why? Because fat is the most calorie dense food and having a storable, high calorie compact energy source would be important to survival. The nature of its fat also made it an important trade good. Like salmon, ooligan returns to its birth stream after years at sea. Its arrival in the early spring made it the first fresh food of the year. In the Tsimshianic languages the arrival of the ooligan … was traditionally announced with the cry, ‘Hlaa aat’ixshi halimootxw!’ … meaning ‘Our Saviour has just arrived!’ As you learned above all fats are hydrophobic (water hating). To isolate the fat, the fish is boiled and the floating fat skimmed off. Ooligan fat composition is 30% saturated fat (like butter) and 55% monounsaturated fat (like plant oils). Importantly it is a solid grease at room temperature. Because it is low in polyunsaturated fats (which oxidize and spoil quickly) it can be stored for later use and used as a trade item. Its composition is said to make it as healthy as olive oil, or better as it has omega 3 fatty acids that reduce risk for diabetes and stroke. It also is rich in three fat soluble vitamins A, E and K. Steroids and Waxes Unlike the phospholipids and fats discussed earlier, steroids have a ring structure. Although they do not resemble other lipids, they are grouped with them because they are also hydrophobic. All steroids have four, linked carbon rings and several of them, like cholesterol, have a short tail. Cholesterol is a steroid. Cholesterol is mainly synthesized in the liver and is the precursor of many steroid hormones, such as testosterone and estradiol. It is also the precursor of vitamins E and K. Cholesterol is the precursor of bile salts, which help in the breakdown of fats and their subsequent absorption by cells. Although cholesterol is often spoken of in negative terms, it is necessary for the proper functioning of the body. It is a key component of the plasma membranes of animal cells. Waxes are made up of a hydrocarbon chain with an alcohol (–OH) group and a fatty acid. Examples of animal waxes include beeswax and lanolin. Plants also have waxes, such as the coating on their leaves, that helps prevent them from drying out. Concept in Action
For an additional perspective on lipids, explore “Biomolecules: The Lipids” through this interactive animation.
Proteins Proteins are one of the most abundant organic molecules in living systems and have the most diverse range of functions of all macromolecules. Proteins may be structural, regulatory, contractile, or protective; they may serve in transport, storage, or membranes; or they may be toxins or enzymes. Each cell in a living system may contain thousands of different proteins, each with a unique function. Their structures, like their functions, vary greatly. They are all, however, polymers of amino acids, arranged in a linear sequence. The functions of proteins are very diverse because there are 20 different chemically distinct amino acids that form long chains, and the amino acids can be in any order. For example, proteins can function as enzymes or hormones. Enzymes, which are produced by living cells, are catalysts in biochemical reactions (like digestion) and are usually proteins. Each enzyme is specific for the substrate (a reactant that binds to an enzyme) upon which it acts. Enzymes can function to break molecular bonds, to rearrange bonds, or to form new bonds. An example of an enzyme is salivary amylase, which breaks down amylose, a component of starch. Hormones are chemical signaling molecules, usually proteins or steroids, secreted by an endocrine gland or group of endocrine cells that act to control or regulate specific physiological processes, including growth, development, metabolism, and reproduction. For example, insulin is a protein hormone that maintains blood glucose levels. Proteins have different shapes and molecular weights; some proteins are globular in shape whereas others are fibrous in nature. For example, hemoglobin is a globular protein, but collagen, found in our skin, is a fibrous protein. Protein shape is critical to its function. Changes in temperature, pH, and exposure to chemicals may lead to permanent changes in the shape of the protein, leading to a loss of function or denaturation (to be discussed in more detail later). All proteins are made up of different arrangements of the same 20 kinds of amino acids. Amino acids are the monomers that make up proteins. Each amino acid has the same fundamental structure, which consists of a central carbon atom bonded to an amino group (–NH 2 ), a carboxyl group (–COOH), and a hydrogen atom. Every amino acid also has another variable atom or group of atoms bonded to the central carbon atom known as the R group. The R group is the only difference in structure between the 20 amino acids; otherwise, the amino acids are identical. The chemical nature of the R group determines the chemical nature of the amino acid within its protein (that is, whether it is acidic, basic, polar, or nonpolar). The sequence and number of amino acids ultimately determine a protein’s shape, size, and function. Each amino acid is attached to another amino acid by a covalent bond, known as a peptide bond, which is formed by a dehydration reaction. The carboxyl group of one amino acid and the amino group of a second amino acid combine, releasing a water molecule. The resulting bond is the peptide bond. The products formed by such a linkage are called polypeptides. While the terms polypeptide and protein are sometimes used interchangeably, a polypeptide is technically a polymer of amino acids, whereas the term protein is used for a polypeptide or polypeptides that have combined together, have a distinct shape, and have a unique function. Evolution in Action The Evolutionary Significance of Cytochrome cCytochrome c is an important component of the molecular machinery that harvests energy from glucose. Because this protein’s role in producing cellular energy is crucial, it has changed very little over millions of years. Protein sequencing has shown that there is a considerable amount of sequence similarity among cytochrome c molecules of different species; evolutionary relationships can be assessed by measuring the similarities or differences among various species’ protein sequences. For example, scientists have determined that human cytochrome c contains 104 amino acids. For each cytochrome c molecule that has been sequenced to date from different organisms, 37 of these amino acids appear in the same position in each cytochrome c. This indicates that all of these organisms are descended from a common ancestor. On comparing the human and chimpanzee protein sequences, no sequence difference was found. When human and rhesus monkey sequences were compared, a single difference was found in one amino acid. In contrast, human-to-yeast comparisons show a difference in 44 amino acids, suggesting that humans and chimpanzees have a more recent common ancestor than humans and the rhesus monkey, or humans and yeast. Protein Structure As discussed earlier, the shape of a protein is critical to its function. To understand how the protein gets its final shape or conformation, we need to understand the four levels of protein structure: primary, secondary, tertiary, and quaternary. The unique sequence and number of amino acids in a polypeptide chain is its primary structure. The unique sequence for every protein is ultimately determined by the gene that encodes the protein. Any change in the gene sequence may lead to a different amino acid being added to the polypeptide chain, causing a change in protein structure and function. In sickle cell anemia, the hemoglobin β chain has a single amino acid substitution, causing a change in both the structure and function of the protein. What is most remarkable to consider is that a hemoglobin molecule is made up of two alpha chains and two beta chains that each consist of about 150 amino acids. The molecule, therefore, has about 600 amino acids. The structural difference between a normal hemoglobin molecule and a sickle cell molecule—that dramatically decreases life expectancy in the affected individuals—is a single amino acid of the 600. Because of this change of one amino acid in the chain, the normally biconcave, or disc-shaped, red blood cells assume a crescent or “sickle” shape, which clogs arteries. This can lead to a myriad of serious health problems, such as breathlessness, dizziness, headaches, and abdominal pain for those who have this disease. Folding patterns resulting from interactions between the non-R group portions of amino acids give rise to the secondary structure of the protein. The most common are the alpha (α)-helix and beta (β)-pleated sheet structures. Both structures are held in shape by hydrogen bonds. In the alpha helix, the bonds form between every fourth amino acid and cause a twist in the amino acid chain. In the β-pleated sheet, the “pleats” are formed by hydrogen bonding between atoms on the backbone of the polypeptide chain. The R groups are attached to the carbons, and extend above and below the folds of the pleat. The pleated segments align parallel to each other, and hydrogen bonds form between the same pairs of atoms on each of the aligned amino acids. The α-helix and β-pleated sheet structures are found in many globular and fibrous proteins. The unique three-dimensional structure of a polypeptide is known as its tertiary structure. This structure is caused by chemical interactions between various amino acids and regions of the polypeptide. Primarily, the interactions among R groups create the complex three-dimensional tertiary structure of a protein. There may be ionic bonds formed between R groups on different amino acids, or hydrogen bonding beyond that involved in the secondary structure. When protein folding takes place, the hydrophobic R groups of nonpolar amino acids lay in the interior of the protein, whereas the hydrophilic R groups lay on the outside. The former types of interactions are also known as hydrophobic interactions. In nature, some proteins are formed from several polypeptides, also known as subunits, and the interaction of these subunits forms the quaternary structure. Weak interactions between the subunits help to stabilize the overall structure. For example, hemoglobin is a combination of four polypeptide subunits. Each protein has its own unique sequence and shape held together by chemical interactions. If the protein is subject to changes in temperature, pH, or exposure to chemicals, the protein structure may change, losing its shape in what is known as denaturation as discussed earlier. Denaturation is often reversible because the primary structure is preserved if the denaturing agent is removed, allowing the protein to resume its function. Sometimes denaturation is irreversible, leading to a loss of function. One example of protein denaturation can be seen when an egg is fried or boiled. The albumin protein in the liquid egg white is denatured when placed in a hot pan, changing from a clear substance to an opaque white substance. Not all proteins are denatured at high temperatures; for instance, bacteria that survive in hot springs have proteins that are adapted to function at those temperatures. Concept in Action For an additional perspective on proteins, explore “Biomolecules: The Proteins” through this interactive animation.
Nucleic Acids Nucleic acids are key macromolecules in the continuity of life. They carry the genetic blueprint of a cell and carry instructions for the functioning of the cell. The two main types of nucleic acids are deoxyribonucleic acid (DNA) and ribonucleic acid (RNA). DNA is the genetic material found in all living organisms, ranging from single-celled bacteria to multicellular mammals. The other type of nucleic acid, RNA, is mostly involved in protein synthesis. The DNA molecules never leave the nucleus, but instead use an RNA intermediary to communicate with the rest of the cell. Other types of RNA are also involved in protein synthesis and its regulation. DNA and RNA are made up of monomers known as nucleotides. The nucleotides combine with each other to form a polynucleotide, DNA or RNA. Each nucleotide is made up of three components: a nitrogenous base, a pentose (five-carbon) sugar, and a phosphate group . Each nitrogenous base in a nucleotide is attached to a sugar molecule, which is attached to a phosphate group.
DNA Double-Helical Structure DNA has a double-helical structure. It is composed of two strands, or polymers, of nucleotides. The strands are formed with bonds between phosphate and sugar groups of adjacent nucleotides. The strands are bonded to each other at their bases with hydrogen bonds, and the strands coil about each other along their length, hence the “double helix” description, which means a double spiral. The alternating sugar and phosphate groups lie on the outside of each strand, forming the backbone of the DNA. The nitrogenous bases are stacked in the interior, like the steps of a staircase, and these bases pair; the pairs are bound to each other by hydrogen bonds. The bases pair in such a way that the distance between the backbones of the two strands is the same all along the molecule. The rule is that nucleotide A pairs with nucleotide T, and G with C, see section 9.1 for more details.
Biological Polymers: Proteins, Carbohydrates, Lipids
Biological polymers are large molecules composed of many similar smaller molecules linked together in a chain-like fashion. The individual smaller molecules are called monomers. When small organic molecules are joined together, they can form giant molecules or polymers. These giant molecules are also called macromolecules. Natural polymers are used to build tissue and other components in living organisms.
Generally speaking, all macromolecules are produced from a small set of about 50 monomers. Different macromolecules vary because of the arrangement of these monomers. By varying the sequence, an incredibly large variety of macromolecules can be produced. While polymers are responsible for the molecular “uniqueness” of an organism, the common monomers are nearly universal.
The variation in the form of macromolecules is largely responsible for molecular diversity. Much of the variation that occurs both within an organism and among organisms can ultimately be traced to differences in macromolecules. Macromolecules can vary from cell to cell in the same organism, as well as from one species to the next.
Biopolymer
Polymer produced by a living organism
“Biopolymers” redirects here. For the scientific journal, see Biopolymers (journal)
Not to be confused with bioplastics , usually semi-synthetic polymers produced from renewable biomass sources.
Biopolymers are natural polymers produced by the cells of living organisms. Biopolymers consist of monomeric units that are covalently bonded to form larger molecules. There are three main classes of biopolymers, classified according to the monomers used and the structure of the biopolymer formed: polynucleotides, polypeptides, and polysaccharides. Polynucleotides, such as RNA and DNA, are long polymers composed of 13 or more[clarification needed] nucleotide monomers. Polypeptides and proteins, are polymers of amino acids and some major examples include collagen, actin, and fibrin. Polysaccharides are linear or branched polymeric carbohydrates and examples include starch, cellulose and alginate. Other examples of biopolymers include natural rubbers (polymers of isoprene), suberin and lignin (complex polyphenolic polymers), cutin and cutan (complex polymers of long-chain fatty acids) and melanin.
Biopolymers have applications in many fields including the food industry, manufacturing, packaging, and biomedical engineering.[1]
Biopolymers versus synthetic polymers [ edit ]
A major defining difference between biopolymers and synthetic polymers can be found in their structures. All polymers are made of repetitive units called monomers. Biopolymers often have a well-defined structure, though this is not a defining characteristic (example: lignocellulose): The exact chemical composition and the sequence in which these units are arranged is called the primary structure, in the case of proteins. Many biopolymers spontaneously fold into characteristic compact shapes (see also “protein folding” as well as secondary structure and tertiary structure), which determine their biological functions and depend in a complicated way on their primary structures. Structural biology is the study of the structural properties of biopolymers. In contrast, most synthetic polymers’ have much simpler and more random (or stochastic) structures. This fact leads to a molecular mass distribution that is missing in biopolymers. In fact, as their synthesis is controlled by a template-directed process in most in vivo systems, all biopolymers of a type (say one specific protein) are all alike: they all contain similar sequences and numbers of monomers and thus all have the same mass. This phenomenon is called monodispersity in contrast to the polydispersity encountered in synthetic polymers. As a result, biopolymers have a dispersity of 1.[2]
Conventions and nomenclature [ edit ]
Polypeptides [ edit ]
The convention for a polypeptide is to list its constituent amino acid residues as they occur from the amino terminus to the carboxylic acid terminus. The amino acid residues are always joined by peptide bonds. Protein, though used colloquially to refer to any polypeptide, refers to larger or fully functional forms and can consist of several polypeptide chains as well as single chains. Proteins can also be modified to include non-peptide components, such as saccharide chains and lipids.
Nucleic acids [ edit ]
The convention for a nucleic acid sequence is to list the nucleotides as they occur from the 5′ end to the 3′ end of the polymer chain, where 5′ and 3′ refer to the numbering of carbons around the ribose ring which participate in forming the phosphate diester linkages of the chain. Such a sequence is called the primary structure of the biopolymer.
Sugar [ edit ]
Sugar polymers can be linear or branched and are typically joined with glycosidic bonds. The exact placement of the linkage can vary, and the orientation of the linking functional groups is also important, resulting in α- and β-glycosidic bonds with numbering definitive of the linking carbons’ location in the ring. In addition, many saccharide units can undergo various chemical modifications, such as amination, and can even form parts of other molecules, such as glycoproteins.
Structural characterization [ edit ]
There are a number of biophysical techniques for determining sequence information. Protein sequence can be determined by Edman degradation, in which the N-terminal residues are hydrolyzed from the chain one at a time, derivatized, and then identified. Mass spectrometer techniques can also be used. Nucleic acid sequence can be determined using gel electrophoresis and capillary electrophoresis. Lastly, mechanical properties of these biopolymers can often be measured using optical tweezers or atomic force microscopy. Dual-polarization interferometry can be used to measure the conformational changes or self-assembly of these materials when stimulated by pH, temperature, ionic strength or other binding partners.
Common biopolymers [ edit ]
Collagen:[3] Collagen is the primary structure of vertebrates and is the most abundant protein in mammals. Because of this, collagen is one of the most easily attainable biopolymers, and used for many research purposes. Because of its mechanical structure, collagen has high tensile strength and is a non toxic, easily absorbable, biodegradable and biocompatible material. Therefore, it has been used for many medical applications such as in treatment for tissue infection, drug delivery systems, and gene therapy.
Silk fibroin:[4] Silk Fibroin (SF) is another protein rich biopolymer that can be obtained from different silk worm species, such as the mulberry worm Bombyx mori. In contrast to collagen, SF has a lower tensile strength but has strong adhesive properties due to its insoluble and fibrous protein composition. In recent studies, silk fibroin has been found to possess anticoagulation properties and platelet adhesion. Silk fibroin has been additionally found to support stem cell proliferation in vitro.
Gelatin: Gelatin is obtained from type I collagen consisting of cysteine, and produced by the partial hydrolysis of collagen from bones, tissues and skin of animals.[5] There are two types of gelatin, Type A and Type B. Type A collagen is derived by acid hydrolysis of collagen and has 18.5% nitrogen. Type B is derived by alkaline hydrolysis containing 18% nitrogen and no amide groups. Elevated temperatures cause the gelatin to melts and exists as coils, whereas lower temperatures result in coil to helix transformation. Gelatin contains many functional groups like NH2, SH, and COOH which allow for gelatin to be modified using nanoparticles and biomolecules. Gelatin is an Extracellular Matrix protein which allows it to be applied for applications such as wound dressings, drug delivery and gene transfection.[5]
Starch: Starch is an inexpensive biodegradable biopolymer and copious in supply. Nanofibers and microfibers can be added to the polymer matrix to increase the mechanical properties of starch improving elasticity and strength. Without the fibers, starch has poor mechanical properties due to its sensitivity to moisture. Starch being biodegradable and renewable is used for many applications including plastics and pharmaceutical tablets.
Cellulose: Cellulose is very structured with stacked chains that result in stability and strength. The strength and stability comes from the straighter shape of cellulose caused by glucose monomers joined together by glycogen bonds. The straight shape allows the molecules to pack closely. Cellulose is very common in application due to its abundant supply, its biocompatibility, and is environmentally friendly. Cellulose is used vastly in the form of nano-fibrils called nano-cellulose. Nano-cellulose presented at low concentrations produces a transparent gel material. This material can be used for biodegradable, homogeneous, dense films that are very useful in the biomedical field.
Alginate: Alginate is the most copious marine natural polymer derived from brown seaweed. Alginate biopolymer applications range from packaging, textile and food industry to biomedical and chemical engineering. The first ever application of alginate was in the form of wound dressing, where its gel-like and absorbent properties were discovered. When applied to wounds, alginate produces a protective gel layer that is optimal for healing and tissue regeneration, and keeps a stable temperature environment. Additionally, there have been developments with alginate as a drug delivery medium, as drug release rate can easily be manipulated due to a variety of alginate densities and fibrous composition.
Biopolymer applications [ edit ]
The applications of biopolymers can be categorized under two main fields, which differ due to their biomedical and industrial use.[1]
Biomedical [ edit ]
Because one of the main purposes for biomedical engineering is to mimic body parts to sustain normal body functions, due to their biocompatible properties, biopolymers are used vastly for tissue engineering, medical devices and the pharmaceutical industry.[3] Many biopolymers can be used for regenerative medicine, tissue engineering, drug delivery, and overall medical applications due to their mechanical properties. They provide characteristics like wound healing, and catalysis of bio-activity, and non-toxicity.[6] Compared to synthetic polymers, which can present various disadvantages like immunogenic rejection and toxicity after degradation, many biopolymers are normally better with bodily integration as they also possess more complex structures, similar to the human body.
More specifically, polypeptides like collagen and silk, are biocompatible materials that are being used in ground breaking research, as these are inexpensive and easily attainable materials. Gelatin polymer is often used on dressing wounds where it acts as an adhesive. Scaffolds and films with gelatin allow for the scaffolds to hold drugs and other nutrients that can be used to supply to a wound for healing.
As collagen is one of the more popular biopolymer used in biomedical science, here are some examples of their use:
Collagen based drug delivery systems: collagen films act like a barrier membrane and are used to treat tissue infections like infected corneal tissue or liver cancer.[7] Collagen films have all been used for gene delivery carriers which can promote bone formation.
Collagen sponges: Collagen sponges are used as a dressing to treat burn victims and other serious wounds. Collagen based implants are used for cultured skin cells or drug carriers that are used for burn wounds and replacing skin.[7]
Collagen as haemostat: When collagen interacts with platelets it causes a rapid coagulation of blood. This rapid coagulation produces a temporary framework so the fibrous stroma can be regenerated by host cells. Collagen based haemostat reduces blood loss in tissues and helps manage bleeding in cellular organs like the liver and spleen.
Chitosan is another popular biopolymer in biomedical research.[according to whom?] Chitosan is derived from chitin, the main component in the exoskeleton of crustaceans and insects and the second most abundant biopolymer in the world.[3] Chitosan has many excellent characteristics for biomedical science. Chitosan is biocompatible, it is highly bioactive, meaning it stimulates a beneficial response from the body, it can biodegrade which can eliminate a second surgery in implant applications, can form gels and films, and is selectively permeable. These properties allow for various biomedical applications of chitosan.
Chitosan as drug delivery: Chitosan is used mainly with drug targeting because it has potential to improve drug absorption and stability. In addition, chitosan conjugated with anticancer agents can also produce better anticancer effects by causing gradual release of free drug into cancerous tissue.
Chitosan as an anti-microbial agent: Chitosan is used to stop the growth of microorganisms. It performs antimicrobial functions in microorganisms like algae, fungi, bacteria, and gram-positive bacteria bacteria of different yeast species.
Chitosan composite for tissue engineering: Blended powder of chitosan along with alginate are used together to form functional wound dressings. These dressings create a moist environment which aids in the healing process. This wound dressing is also very biocompatible, biodegradable and has porous structures that allows cells to grow into the dressing.[3]
Industrial [ edit ]
Food: Biopolymers are being used in the food industry for things like packaging, edible encapsulation films and coating foods. Polylactic acid (PLA) is very common in the food industry due to is clear color and resistance to water. However, most polymers have a hydrophilic nature and start deteriorating when exposed to moisture. Biopolymers are also being used as edible films that encapsulate foods. These films can carry things like antioxidants, enzymes, probiotics, minerals, and vitamins. The food consumed encapsulated with the biopolymer film can supply these things to the body.
Packaging: The most common biopolymers used in packaging are polyhydroxyalkanoate (PHA), polylactic acid (PLA), and starch. Starch and PLA are commercially available and biodegradable, making them a common choice for packaging. However, their barrier properties and thermal properties are not ideal. Hydrophilic polymers are not water resistant and allow water to get through the packaging which can affect the contents of the package. Polyglycolic acid (PGA) is a biopolymer that has great barrier characteristics and is now being used to correct the barrier obstacles from PLA and starch.
Water purification: Chitosan has been used for water purification. It is used as a flocculant that only takes a few weeks or months rather than years to degrade into the environment. Chitosan purifies water by chelation. This is the process in which binding sites along the polymer chain bind with the metal in the water forming chelates. Chitosan has been shown to be an excellent candidate for use in storm and waste water treatment.[8]
As materials [ edit ]
Some biopolymers- such as PLA, naturally occurring zein, and poly-3-hydroxybutyrate can be used as plastics, replacing the need for polystyrene or polyethylene based plastics.
Some plastics are now referred to as being ‘degradable’, ‘oxy-degradable’ or ‘UV-degradable’. This means that they break down when exposed to light or air, but these plastics are still primarily (as much as 98 per cent) oil-based and are not currently certified as ‘biodegradable’ under the European Union directive on Packaging and Packaging Waste (94/62/EC). Biopolymers will break down, and some are suitable for domestic composting.[9]
Biopolymers (also called renewable polymers) are produced from biomass for use in the packaging industry. Biomass comes from crops such as sugar beet, potatoes or wheat: when used to produce biopolymers, these are classified as non food crops. These can be converted in the following pathways:
Sugar beet > Glyconic acid > Polyglyconic acid
Starch > (fermentation) > Lactic acid > Polylactic acid (PLA)
Biomass > (fermentation) > Bioethanol > Ethene > Polyethylene
Many types of packaging can be made from biopolymers: food trays, blown starch pellets for shipping fragile goods, thin films for wrapping.
Environmental impacts [ edit ]
Biopolymers can be sustainable, carbon neutral and are always renewable, because they are made from plant or animal materials which can be grown indefinitely. Since these materials come from agricultural crops, their use could create a sustainable industry. In contrast, the feedstocks for polymers derived from petrochemicals will eventually deplete. In addition, biopolymers have the potential to cut carbon emissions and reduce CO 2 quantities in the atmosphere: this is because the CO 2 released when they degrade can be reabsorbed by crops grown to replace them: this makes them close to carbon neutral.
Some biopolymers are biodegradable: they are broken down into CO 2 and water by microorganisms. Some of these biodegradable biopolymers are compostable: they can be put into an industrial composting process and will break down by 90% within six months. Biopolymers that do this can be marked with a ‘compostable’ symbol, under European Standard EN 13432 (2000). Packaging marked with this symbol can be put into industrial composting processes and will break down within six months or less. An example of a compostable polymer is PLA film under 20μm thick: films which are thicker than that do not qualify as compostable, even though they are “biodegradable”.[10] In Europe there is a home composting standard and associated logo that enables consumers to identify and dispose of packaging in their compost heap.[9]
See also [ edit ]
Which class of biological polymer has the greatest functional variety? 1) DNA 2) polysaccharides 3) proteins 4) RNA
Organic molecules are comprised of carbon atoms that are covalently bonded with other atoms, such as hydrogen. Learn about organic molecules, the difference between saturated and unsaturated molecules, and how carbon and hydrogen can form different combinations of molecules.
Understand how big a molecule is and explore types of molecules. Discover how they relate to living things and what the most common molecules in living things are.
Learn about natural polymers. Understand what natural polymers are, explore their types, and discover some important natural polymers examples in everyday life.
Learn an organic molecule definition and see examples. Explore functional groups and their role in organic molecules, and see why organic molecules are important.
Explore carbon-containing compounds and what the study of substances that contain carbon is called. Learn what makes carbon unique and why it is essential to life.
Learn about polymers, their properties, their examples, and their applications in life. See their products, what they are made of, and how they are each made.
Explore copolymers. Learn the definition of a copolymer and understand its properties. Discover the different types of copolymers with various examples.
Explore the 6 elements of life. Discover what the 6 elements of life are, understand what CHNOPS elements are, and explore their roles in living organisms.
Six Elements of Life (CHNOPS) Overview & Roles | What is CHNOPS?
Gain an understanding of DNA polymerase and RNA polymerase. Learn what DNA polymerase does and explore the functions of RNA primase and their end products.
Explore the ovarian cycle phases, their definition, and stages like puberty and menopause. Discover how ovarian cycle events occur and the phases of reproduction.
Ovarian Cycle Phases Overview & Parts | What are the Stages of the Ovarian Cycle?
Learn the definition of silent mutation. Understand the causes of silent mutation and its clinical implications, including examples.
Learn about synthetic polymers and their structure. Understand what synthetic polymers are and discover examples of polymers commonly used in our synthetic clothing.
Learn about functional groups. Understand what functional groups are, and explore the functional groups list, including alkyl, ester, and aldehyde functional group.
Learn about elements, compounds, and molecules, and the difference between molecules and compounds. Understand how elements and the number of atoms relate to molecules and compounds.
Learn about the pericardium and the three layers of the heart wall. Discover distinctive features of the pericardium, epicardium, myocardium, and endocardium.
The Three Layers of the Heart Wall | Location, Structure & Function
Explore carbon structure, understand what a carbon skeleton is, how to draw it, and what it looks like. Explain the importance of carbon skeleton structures.
Cytology Early History | What is Cytology? Learn what cytology is. Understand the early history of cytology and discover how the field has developed over the years with the usage of cytology tests.
Molecular Clock Concept & Use | What is a Molecular Clock? Understand the definition of a molecular clock. Explore how does the molecular clock work and see examples of molecular clocks. Explore the use of molecular clocks.
Systemic Circulation: Overview & Examples | Systemic vs. Pulmonary Circulation Learn the systemic circulation definition. Understand the systemic circuit pathway and learn the difference between pulmonary and systemic circulation.
Which class of biological polymer has the greatest functional variety?
Approximately 32 different monomeric carbohydrate subunits are found in various natural polysaccharides. Proteins are composed of 20 different amino acids. DNA and RNA are each synthesized from four nucleotides. Which class of biological polymer has the greatest functional variety?
3)proteins
Combinations of 20 building blocks with very different properties makes for a great variation in higher order function. The secondary local patterns that produce the tertiary 3D shape in space result from this primary properties in combination. Then proteins can be combined as subunits for further variation in function. This is how proteins can become catalytic in so many ways.
3)proteins – eg. enzymes, antibodies, receptors, etc….
Which class of biological polymer has the greatest functional variety
Ask a Question
How to use? Just show us this coupon on a screen
Quick Contact to Help?
For any Query regarding a website or any issue please contact with us we are here for solving your problem 24/7
Chapter 5 Flashcards
45
The R group or side chain of the amino acid serine is –CH₂–OH. The R group or side chain of the amino acid leucine is –CH₂–CH–(CH₃)₂. Where would you expect to find these amino acids in a globular protein in aqueous solution?
A) Serine would be in the interior, and leucine would be on the exterior of the globular protein.
B) Leucine would be in the interior, and serine would be on the exterior of the globular protein.
C) Both serine and leucine would be in the interior of the globular protein.
D) Both serine and leucine would be on the exterior of the globular protein.
E) Both serine and leucine would be in the interior and on the exterior of the globular protein.
What are the 4 biological polymers? – Book Vea
Which class of biological macromolecules has the greatest functional variety?
Proteins. Proteins are one of the most abundant organic molecules in living systems and have the most diverse range of functions of all macromolecules. Proteins may be structural, regulatory, contractile, or protective; they may serve in transport, storage, or membranes; or they may be toxins or enzymes.
What are the 4 biological polymers?
Proteins, carbohydrates, nucleic acids, and lipids are the four major classes of biological macromoleculeslarge molecules necessary for life that are built from smaller organic molecules. Macromolecules are made up of single units known as monomers that are joined by covalent bonds to form larger polymers.
What are the main biological polymers?
There are four basic kinds of biological macromolecules: carbohydrates, lipids, proteins, and nucleic acids. These polymers are composed of different monomers and serve different functions.
What is the most common biological molecule?
Water is the most abundant molecule in cells, accounting for 70% or more of total cell mass. Consequently, the interactions between water and the other constituents of cells are of central importance in biological chemistry.
Which biological polymer has the greatest functional variety?
Protein has protein have greatest functional yeah, greatest the greatest functional functional variety.
What are the 4 types of macromolecules and their functions?
There are four major classes of biological macromolecules:
carbohydrates.
lipids.
proteins.
nucleic acids.
5 Mar 2021
What are the major classes of biological macromolecules?
TermMeaningBiological macromoleculeA large, organic molecule such as carbohydrates, lipids, proteins, and nucleic acids.MonomerA molecule that is a building block for larger molecules (polymers). For example, an amino acid acts as the building blocks for proteins.1 more row
What are the 4 types of biological molecules and their monomers and polymers?
There are four basic kinds of biological macromolecules: carbohydrates, lipids, proteins, and nucleic acids. These polymers are composed of different monomers and serve different functions.
What are the 4 major biological molecules?
As wex26#39;ve learned, there are four major classes of biological macromolecules:
Proteins (polymers of amino acids)
Carbohydrates (polymers of sugars)
Lipids (polymers of lipid monomers)
Nucleic acids (DNA and RNA; polymers of nucleotides)
What are the four biological monomers?
Biomolecules have a wide range of sizes and structures and perform a vast array of functions. The four major types of biomolecules are carbohydrates, lipids, nucleic acids, and proteins
What are the 4 major biological polymers?
As wex26#39;ve learned, there are four major classes of biological macromolecules:
Proteins (polymers of amino acids)
Carbohydrates (polymers of sugars)
Lipids (polymers of lipid monomers)
Nucleic acids (DNA and RNA; polymers of nucleotides)
What are the three major biological polymers?
There are three main classes of biopolymers, classified according to the monomers used and the structure of the biopolymer formed: polynucleotides, polypeptides, and polysaccharides. Polynucleotides, such as RNA and DNA, are long polymers composed of 13 or more nucleotide monomers.
What is the most important biological molecule?
Nucleic acids are the most important macromolecules for the continuity of life. They carry the genetic blueprint of a cell and carry instructions for the functioning of the cell.
What are the four most common biological atoms?
The four most abundant elements in living matter (C, N, O, and H) have low atomic numbers and are thus light elements capable of forming strong bonds with other atoms to produce molecules (Figure 2.3. 4). Unlike carbon, nitrogen forms up to three bonds, oxygen forms up to two, and hydrogen forms one.
Which class of biological molecules has the greatest functional variety?
Proteins are one of the most abundant organic molecules in living systems and have the most diverse range of functions of all macromolecules.
Which of the biological polymers has the most diverse functions?
Proteins
What are the 4 types of biological polymers?
Four Classes of Biological Macromolecules carbohydrates. lipidsproteinsnucleic acids
What are the functions of macromolecules?
For example, macromolecules provide structural support, a source of stored fuel, the ability to store and retrieve genetic information, and the ability to speed biochemical reactions. Four major types of macromoleculesproteins, carbohydrates, nucleic acids, and lipidsplay these important roles in the life of a cell.
What are the 4 main macromolecules?
11.1 Introduction: The Four Major Macromolecules These are the carbohydrates, lipids (or fats), proteins, and nucleic acids. All of the major macromolecule classes are similar, in that, they are large polymers that are assembled from small repeating monomer subunits.
What are the four classes of biological macromolecules?
There are four major classes of biological macromolecules:
carbohydrates.
lipids.
proteins.
nucleic acids.
5 Mar 2021
What is the major class of macromolecules?
11.1 Introduction: The Four Major Macromolecules These are the carbohydrates, lipids (or fats), proteins, and nucleic acids. All of the major macromolecule classes are similar, in that, they are large polymers that are assembled from small repeating monomer subunits.
What are the 4 classes of macromolecules and give a function for each?
Nucleic acids: Stores and transfers info. Carbohydrates; Store energy, provide fuel, and build structure in body, main source of energy, structure of plant cell wall. Lipid: Insulator and stores fat and energy. Protein: Provide structural support,transport, enzymes, movement, defense.
What are the major biological macromolecules?
Biological macromoleculeBuilding blocksCarbohydratesMonosaccharides (simple sugars)LipidsFatty acids and glycerolProteinsAmino acidsNucleic acidsNucleotides
What are the 4 biological molecules and their monomers?
There are four major classes of large biological moleculescarbohydrates, lipids, proteins, and nucleic acids. Carbohydrates are made up of monomers called monosaccharides that contain carbon, oxygen, and hydrogen.
What are the 4 biomolecule polymers and matching monomers?
Four Classes of Biological Macromolecules carbohydrates. lipidsproteinsnucleic acids
키워드에 대한 정보 which class of biological polymer has the greatest functional variety
다음은 Bing에서 which class of biological polymer has the greatest functional variety 주제에 대한 검색 결과입니다. 필요한 경우 더 읽을 수 있습니다.
이 기사는 인터넷의 다양한 출처에서 편집되었습니다. 이 기사가 유용했기를 바랍니다. 이 기사가 유용하다고 생각되면 공유하십시오. 매우 감사합니다!
사람들이 주제에 대해 자주 검색하는 키워드 003-Biological Polymers
- bio polymers
003-Biological #Polymers
YouTube에서 which class of biological polymer has the greatest functional variety 주제의 다른 동영상 보기
주제에 대한 기사를 시청해 주셔서 감사합니다 003-Biological Polymers | which class of biological polymer has the greatest functional variety, 이 기사가 유용하다고 생각되면 공유하십시오, 매우 감사합니다.